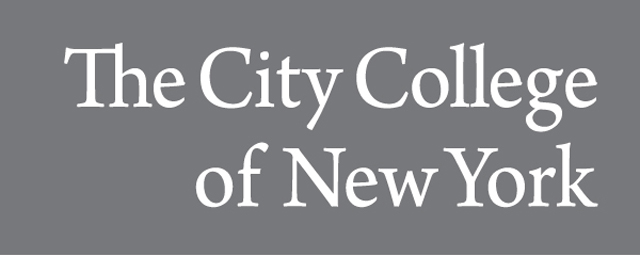
Dissertations and Theses
Date of Award
2023
Document Type
Dissertation
Department
Chemical Engineering
First Advisor
Sanjoy Banerjee
Second Advisor
Robert Messinger
Keywords
zinc oxide, zinc batteries, li-ion batteries, battery failure mechanisms, high-temperature li-ion batteries, low-temperature zinc batteries
Abstract
We are beginning a global transition away from the use of fossil fuels for our energy needs toward using renewable energy sources in order to mitigate the effects of climate change. One of the biggest challenges to this transition is that, unlike fossil fuels, renewable energy systems have inherent intermittency and require supporting energy storage systems to enable reliable power distribution and electrified transportation. Rechargeable batteries are an attractive energy storage option because of their high energy efficiency, versatility, modularity, and ease of use. Lithium-ion batteries (LIBs) have dominated the rechargeable battery market for portable electronics for the past two decades because of their high energy density, power, and cyclability, and they are beginning to be implemented on a large scale for grid-scale energy storage systems and electric vehicles. However, applications for LIBs are limited by their cost, which is influenced by the limited availability of materials used in their construction and by the added cost of control and thermal management systems required for their safe and reliable operation.
Many applications such as renewable energy storage, uninterruptible power supplies, and peak demand shaving require safe and low-cost energy storage systems, thus use of LIBs may not be feasible. One attractive alternative technology is the rechargeable zinc alkaline battery because of its low cost, high energy density, environmental friendliness, inherent safety, and the strategic mineral security of its components. Zinc alkaline batteries have been used in single-discharge applications for a century, however, rechargeable zinc alkaline batteries have not yet been commercialized on a large scale due to limited cyclability at high zinc metal (Zn) utilization, which increases total system cost. The limited cyclability is due in part to the passivation of Zn electrodes by a zinc oxide (ZnO) discharge product that forms under high Zn utilization. Despite decades of research on this ZnO discharge product, its properties and impacts on performance are not fully understood. In this dissertation, the effects of ZnO on Zn battery performance are elucidated, and a thorough study of ZnO electronic properties is presented. Based on this study, techniques are demonstrated for controlling the ZnO conductivity to increase depth of discharge and extend cycle life of Zn alkaline batteries.
Another limitation of many rechargeable batteries is the operating temperature window. Commonly, thermal management systems are implemented to maintain batteries at optimal temperatures. However, these systems add cost and complexity and decrease the overall energy density of an installed system. Expanding the operating temperature of batteries would reduce or eliminate the need for thermal management, which would lower cost and expand the possible applications for rechargeable batteries.
At low temperatures, power and energy density are reduced because of slowed ion diffusion, and at extremely low temperatures, the electrolyte freezes and completely ceases battery operation. In this dissertation, a deep eutectic solvent of an ionic liquid and organic co-solvent is presented, which increases ion diffusivity by orders of magnitude over diffusivity in the pure solvents. Improvements to performance are demonstrated with zinc metal electroplating and stripping, but the principles applied are broadly applicable across battery chemistries.
Although low-cost batteries are needed for future renewable energy storage, for applications where power, energy, and cycle life are critical, such as in many electric vehicles, LIBs are often desired over lower-cost and lower-performance alternatives. Despite the advantages of LIBs, one significant limitation is the upper operating temperature. Temperature limits are commonly imposed to reduce capacity fade resulting in reduced energy density and cycle life. Safe operation at high temperatures would enable higher power discharge and faster charging. Despite extensive research on high-temperature operation, the physical mechanisms for capacity fade are not fully understood. In this dissertation, the mechanisms for capacity fade at high temperature are elucidated and strategies are presented for mitigating capacity fade in next-generation lithium-ion batteries.
In total, this dissertation discusses strategies for improving the performance of different battery chemistries with different advantages and use cases. Although the strategies differ for each chemistry and use case, they are developed in the same way – by first understanding the failure mechanisms under the desired operation conditions. Rechargeable batteries are complex systems that rely on careful control of kinetics, thermodynamics, and transport properties via optimization of materials, operating protocol, and working conditions. By studying these phenomena at different length and time scales, a full understanding of failure mechanisms can be developed, and modifications can be made to battery design and cycling protocol to improve performance. Understanding and controlling failure is the first step toward enabling the next generation of batteries for wide-spread renewable energy storage and electrified transportation.
Recommended Citation
Hawkins, Brendan E., "Understanding Failure Mechanisms using Multi-Scale Analyses to Improve the Performance of Zinc Metal and Lithium-ion Batteries" (2023). CUNY Academic Works.
https://academicworks.cuny.edu/cc_etds_theses/1033